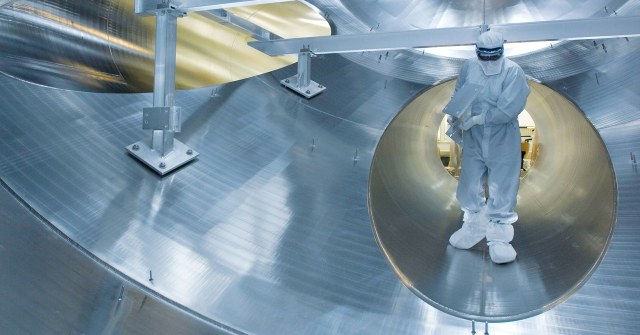
Of all the known particles in the universe, only photons outnumber neutrinos. Despite their abundance, however, neutrinos are hard to catch and inspect, as they interact with matter only very weakly. About 1,000 trillion of the ghostly particles pass through your body every second—with nary a flinch from even a single atom.
Original story reprinted with permission from Quanta Magazine, an editorially independent publication of the Simons Foundation whose mission is to enhance public understanding of science by covering research developments and trends in mathematics and the physical and life sciences.
“The fact that they’re ubiquitous, yet we don’t even know what they weigh, is kind of crazy,” said Deborah Harris, a physicist at the Fermi National Accelerator Laboratory near Chicago and York University in Toronto.
Physicists have long tried to weigh the ghost. And in September, after 18 years of planning, building and calibrating, the Karlsruhe Tritium Neutrino (KATRIN) experiment in southwestern Germany announced its first results: It found that the neutrino can’t weigh more than 1.1 electron-volts (eV), or about one-five-hundred-thousandth the mass of the electron.
This initial estimate, from only one month’s worth of data, improves on previous measurements using similar techniques that placed the upper limit on the neutrino mass at 2 eV. As its data accrues, KATRIN aims to nail the actual mass rather than giving an upper bound.
Why Mass Matters
Mass is one of the most basic and important characteristics of fundamental particles. The neutrino is the only known particle whose mass remains a mystery. Measuring its mass would help point toward new laws of physics beyond the Standard Model, the remarkably successful yet incomplete description for how the universe’s known particles and forces interact. Its measured mass would also serve as a check on cosmologists’ theories for how the universe evolved.
“Depending on what the mass of the neutrino turns out to be, it may lead to very exciting times in cosmology,” said Diana Parno, a physicist at Carnegie Mellon University and a member of the KATRIN team.
Until about two decades ago, neutrinos—which were theoretically predicted in 1930 and discovered in 1956—were presumed to be massless. “When I was in grad school, my textbooks all said neutrinos didn’t have mass,” Harris said.
That changed when, in a discovery that would win the 2015 Nobel Prize, physicists found that neutrinos could morph from one kind to another, oscillating between three “flavor” states: electron, muon and tau. These oscillations can only happen if neutrinos also have three possible mass states, where each flavor has distinct probabilities of being in each of the three mass states. The mass states travel through space differently, so by the time a neutrino goes from point A to point B, this mix of probabilities will have changed, and a detector could measure a different flavor.
If there are three different mass states, then they can’t all be zero—thus, neutrinos have mass. According to recent neutrino oscillation data (which reveals the differences between the mass states rather than their actual values), if the lightest mass state is zero, the heaviest must be at least 0.0495 eV.
Still, that’s so light compared to the mass of other particles that physicists aren’t sure how neutrinos get such tiny masses. Other particles in the Standard Model acquire mass by interacting with the Higgs field, a field of energy that fills all space and drags on massive particles. But for neutrinos, “the mass is so small, you need some additional theory to explain that,” Parno said.
Figuring out how neutrinos acquire mass may resolve other, seemingly related mysteries, such as why there is more matter than antimatter in the universe. Competing theories for the mass-generating mechanism predict different values for the three mass states. While neutrino oscillation experiments have measured the differences between the mass states, experiments like KATRIN home in on a kind of average of the three. Combining the two types of measurements can reveal the value of each mass state, favoring certain theories of neutrino mass over others.
Cosmic Questions
Neutrino mass is also of cosmic importance. Despite their minuscule mass, so many neutrinos were born during the Big Bang that their collective gravity influenced how all the matter in the universe clumped together into stars and galaxies. About a second after the Big Bang, neutrinos were flying around at almost light speed—so fast that they escaped the gravitational pull of other matter. But then they started to slow, which enabled them to help corral atoms, stars and galaxies. The point at which neutrinos began to slow down depends on their mass. Heavier neutrinos would have decelerated sooner and helped make the universe clumpier.
Comments